Both computed tomography and magnetic resonance imaging are considered advanced imaging modalities capable of providing three-dimensional imaging of the distal limb. Magnetic resonance imaging is based on the movement of protons, and it is generally considered to be the modality of choice for imaging the anatomic and physiological properties of a patient (Bushberg et al, 2002). Tissues that have very tightly bound protons, such as normal cortical bone and tendon, appear hypointense (black) on magnetic resonance imaging because the lack of proton movement produces very little signal to generate an image. When soft tissues are damaged, there is fluid accumulation as a result of inflammation; as such, protons can move easily and so injured soft tissue shows up as hyperintense (bright/white) on magnetic resonance imaging, contrasting against the black of normal tendon or ligament. Computed tomography relies on x-ray attenuation and relative tissue density (Bushberg et al, 2002) and is considered the modality of choice for cortical bone.
One of the key differences between computed tomography and magnetic resonance imaging is the time it takes to image the distal limb using each modality. A series of low-field magnetic resonance imaging scans of the foot can take 45–60 minutes to acquire, while a standing computed tomography may take just 2–5 minutes once the patient is sedated and positioned. Most computed tomography scanners image the foot, pastern and fetlock simultaneously with one scan in 2–5 minutes, while imaging these three areas using low-field magnetic resonance imaging would take approxiamtely 3 hours. Additionally, computed tomography exposes the patient to ionising radiation whereas there are no known side effects of magnetic resonance imaging in humans at present (Flanagan and Bell, 2020).
All standing systems are affected by patient motion, including both magnetic resonance imaging and computed tomography. This can be markedly reduced by the use of general anaesthesia; however, general anaesthesia itself does not come without risks in horses (Morgan et al, 2023). Data collected from 6701 general anaesthetics and 1955 standing sedations carried out at 69 centres for multiple reasons, including magnetic resonance imaging, identified a mortality rate of 1% for horses undergoing general anaesthesia and 0.2% for horses undergoing standing sedation (Gozalo-Marcilla et al, 2021). The mortality rate reported in a separate study was 0.6% in horses undergoing magnetic resonance imaging under general anaesthesia. Pyrexia, pneumonia and colic have also been reported in one population following magnetic resonance imaging under general anaesthesia (Morgan et al, 2023).
Available systems for computed tomography imaging
There are many computed tomography machines available from companies such as Siemens, Philips and Canon that have been adapted from use in humans for use in horses. These machines tend to be fan beam systems with medium-bore sizes. Several companies have designed computed tomography machines specifically adapted for horses, to increase the amount of the horse's body that can be physically fitted into the central bore. Computed tomography machines can be divided into cone beam and fan beam, depending on the shape of the x-ray beam they emit and the way they detect the x-rays. Additionally, cone beam systems tend to use less ionising radiation. Qalibra, AstoCT (Brounts et al, 2022), Pegaso, 4DD Equimagine and Hallmarq VisionCT (Figure 1) all provide systems with adaptations for horses. Qalibra and AstoCT are fan beam systems, while Pegaso, 4DD Equimagine and Hallmarq VisionCT are cone beam systems. In general, both cone and fan beam systems can be comparable at the evaluation of bone (if similar slice thicknesses are compared) but cone beam systems are inferior for the evaluation of soft tissues (Stewart et al, 2021; 2023). The Qalibra, AstoCT, 4DD Equimagine and Hallmarq VisionCT are all marketed for their capability to provide computed tomography images of the equine distal limb in the standing sedated horse.

Available systems for magnetic resonance imaging
Magnetic resonance imaging systems can be divided into low field (<1.0T) and high field (>1.5T) depending on the strength of their magnetic field. Magnetic field strength was found to have more of an influence on image quality than if the patient was anaesthetised in a small study of the equine foot (Byrne et al, 2021), although motion in the standing sedated horse is more likely to influence image quality of the fetlock and proximal metacarpal regions.
The most widely available system in the UK is the low-field Hallmarq (Figure 2a) standing magnetic resonance imaging system that can be found in 24 veterinary practices in England and two in Scotland. The Esoate (Figure 2b) is a low-field magnetic resonance imaging system that requires general anaesthesia and is available at two veterinary practices in the UK. There are also high-field magnetic resonance imaging scanners that are adapted from human medicine, such as the Siemens system found at the Royal Veterinary College in London. Magnetic field strength was found to have more of an influence on image quality than if the patient was anaesthetised in a small study of the equine foot (Byrne et al, 2021), although motion in the standing sedated horse is more likely to influence image quality of the fetlock and proximal metacarpal regions.
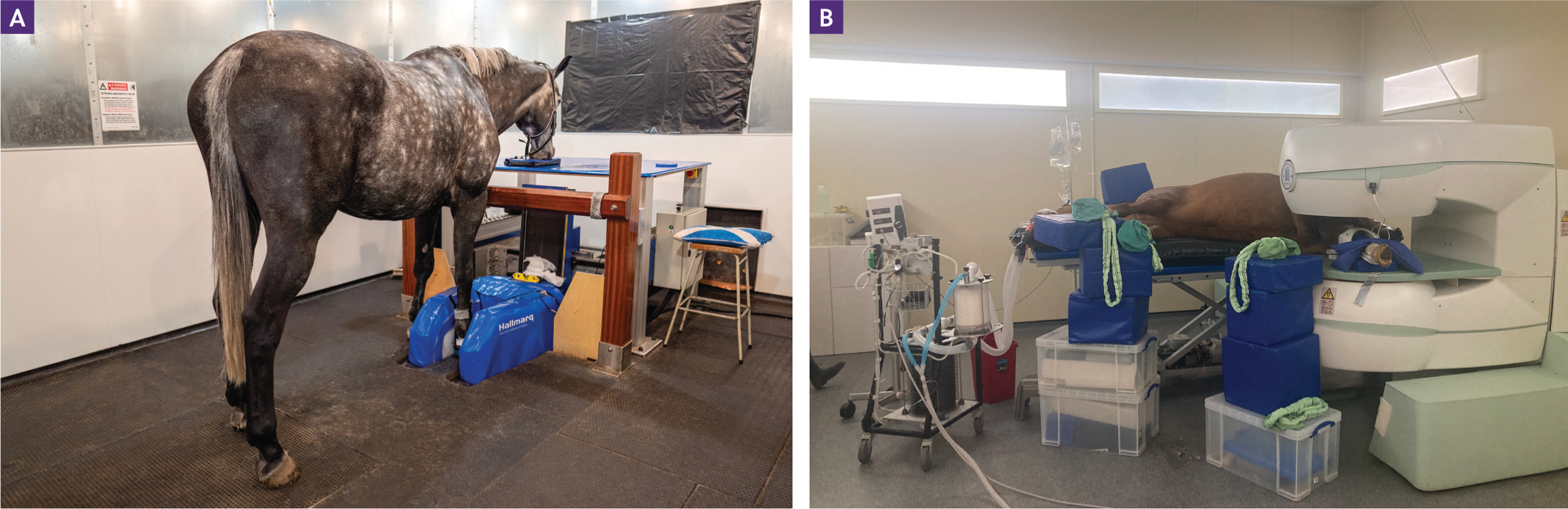
Computed tomography and magnetic resonance imaging by anatomic structure
Bone imaging
Computed tomography is generally regarded as superior to magnetic resonance imaging for the evaluation of cortical bone. Fan beam computed tomography has higher anatomic visualisation scores when evaluating bone margins in the foot in comparison to low-field standing magnetic resonance imaging (Vallance et al, 2012a). Vallance et al (2012a) used a 3 mm slice thickness for computed tomography and 5 mm slice thickness for magnetic resonance imaging sequences, which is likely to have influenced the results of this study (most fan beam computed tomography machines would ideally use slice thicknesses of <1 mm to produce a better quality for reformatted images). Fan beam computed tomography is reportedly superior to both cone beam computed tomography and standing magnetic resonance imaging for the detection of dorsoproximal osteochondral fragments of the proximal phalanx, and cone beam computed tomography was superior to standing magnetic resonance imaging (Lin et al, 2023a).
A prospective clinical study suggested that the use of robotic cone beam computed tomography was an efficient screening tool for evaluation of subchondral bone morphology in the 2-year-old racehorse (Ciamillo et al, 2024). Cone beam computed tomography was found to be superior to fan beam and standing magnetic resonance imaging for the identification of fissures at the parasagittal groove of the third metacarpus and sagittal groove of the proximal phalanx in one cadaver study (Lin et al, 2023b). Direct extrapolation to the clinical patient may not be possible, as motion artefacts are likely to affect the Hallmarq VisionCT system in the sedated horse. Additionally, the fissures evaluated in this work were a few millimetres in length rather than the 5–15 μm that would represent true microcracks. The clinical relevance of focal lesions of the sagittal groove of the proximal phalanx is still highly debated, with one study identifying their presence in untrained yearlings (Nagy et al, 2023).
Both magnetic resonance imaging and computed tomography can detect palmar osteochondral disease in the equine metacarpus, and while cone beam and fan beam computed tomography were both more sensitive than magnetic resonance imaging, the fluid sensitive sequences available with magnetic resonance imaging may allow better assessment of pathological status and severity (Lin et al, 2023c). Magnetic resonance imaging allows the detection of ‘bone oedema’, which is often considered to represent ‘active’ changes (Powell et al, 2010). Currently, dual-energy computed tomography – where two computed tomography images at different kVs are acquired – is capable of detecting bone oedema, but has not been validated in the equine distal limb in clinical cases (Germonpré et al, 2024).
Hoof wall imaging
The hoof wall appears as a region of signal void (black) on low-field magnetic resonance imaging (Figure 3a), whereas the position of the distal phalanx within the hoof capsule can easily be assessed with computed tomography (Figure 3b). One study urged caution when using magnetic resonance imaging because of its limited accuracy for assessment of foot conformation (Bolt et al, 2022). Some authors have reported the use of damp cotton wool to help delineate the keratinised hoof wall; however, the present author finds this can alter the greyscale and prefers not to use cotton wool.

Magnetic resonance imaging has been used in the evaluation of solar penetrations for many years and can provide valuable information on the direction of the penetrating tract, especially if horses are scanned within 7 days of injury (del Junco et al, 2012). Neither computed tomography or magnetic resonance imaging can provide definitive positive detection of synovial infection in every case. Detection of relatively large foreign bodies within the hoof wall was confirmed in one study for both fan beam computed tomography (slicing at 0.5–1 mm) and standing magnetic resonance imaging (slicing at 3–5 mm), with higher sensitivity and specificity for computed tomography (Ogden et al, 2021). The most difficult foreign body to detect in either modality in the cadaveric study was soaked wood, and this is also the author's experience with clinical cases (Figure 4). Both computed tomography- and magnetic resonance imaging-assisted partial hoof wall resection for the removal of keratomas has been described. Getman et al (2011) preferred to use computed tomography because of reduced image acquisition times (Getman et al, 2011). Intra-operative computed tomography has been used to guide lag screw placement for the repair of type II fractures of the distal phalanx (Figure 5) (Smanik et al, 2022).

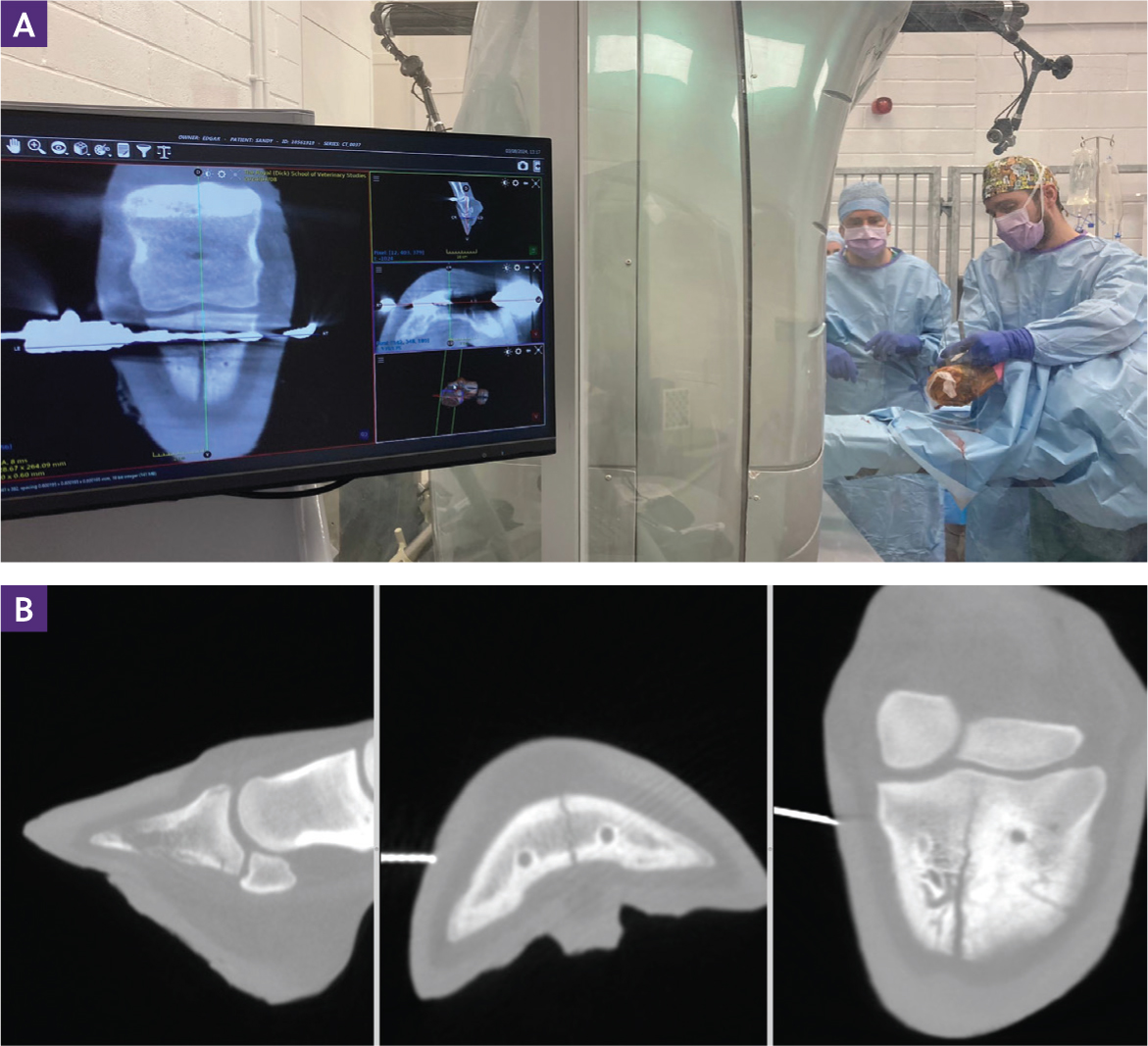
Tendon and ligament imaging
Standing magnetic resonance imaging of the equine distal limb has revolutionised the diagnosis and treatment of tendon injury with-in the hoof capsule (Dyson and Murray, 2007; Barrett et al, 2017) (Figure 6), and has been shown to be more sensitive than computed tomography for insertional injury of the equine deep digital flexor tendon (Vallance et al, 2012b). Soft tissue post-processing algorithms are currently better developed for fan beam systems. The imaging of soft tissues with computed tomography can be improved with contrast enhancement, but this involves the use of a tourniquet and intra-arterial injection of contrast material (Figure 7), such that the contrast material highlights areas of increased vascular permeability. The proximal suspensory insertion is potentially one area in which contrast-enhanced computed tomography may become popular, as it can be difficult to acquire motion-free images of this area in the standing patient. Additionally, high-field magnetic resonance imaging under general anaesthesia is less available than computed tomography under general anaesthesia.
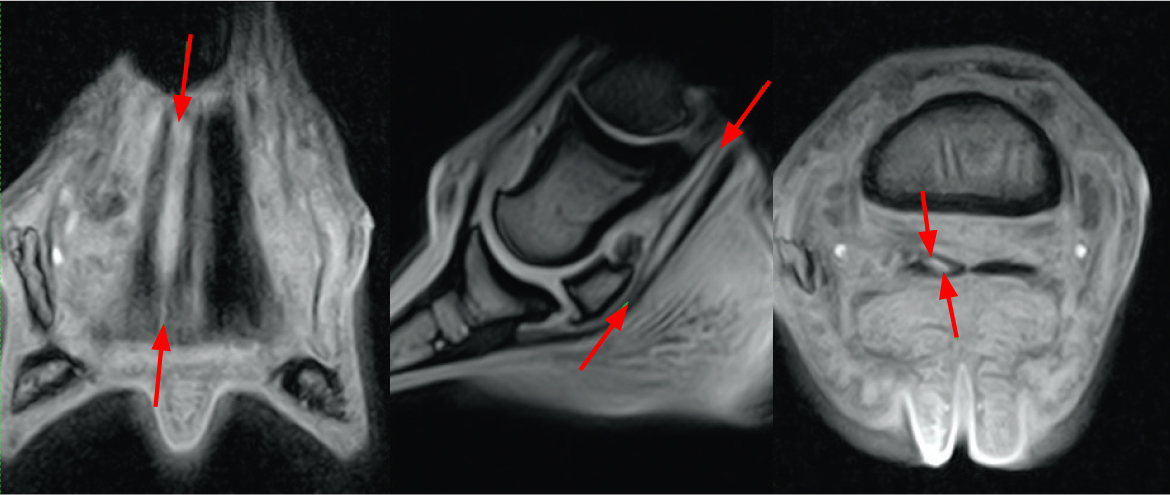
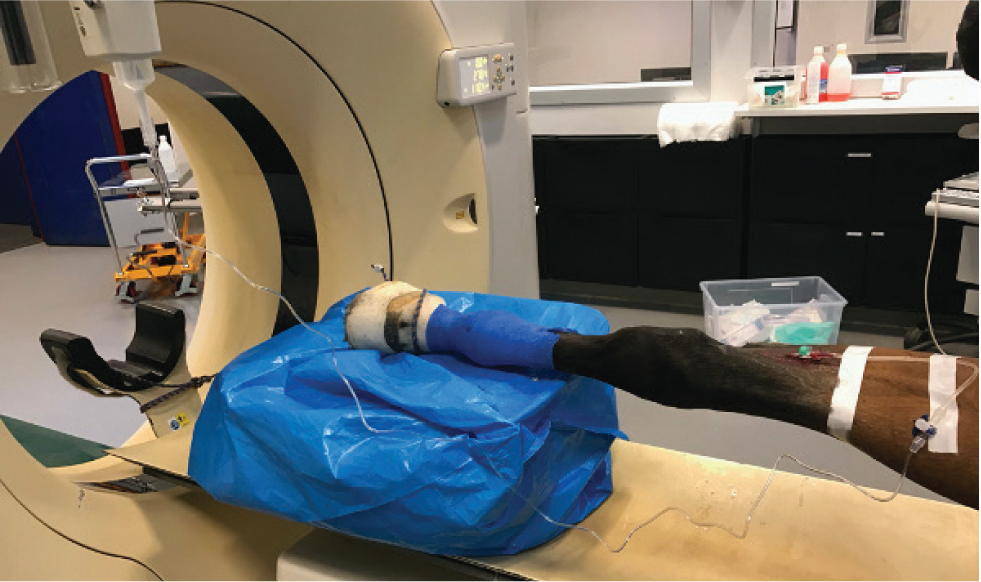
Contrast-enhanced computed tomography of the equine distal limb has been described where all cases underwent a distal limb arteriogram, a distal interphalangeal joint bursogram and a navicular bursogram (Pauwels et al, 2021). Findings were considered comparable to low-field magnetic resonance imaging, but a direct sensitivity and specificity comparison was not made (Pauwels et al, 2021). One area in which computed tomography may be helpful for diagnosing a tendon injury is three-dimensional imaging of the digital flexor tendon sheath to help with the diagnosis of marginal tears of the deep digital flexor tendon and the manica flexoria (Shanklin et al, 2024). As it is a ligamentous structure, imaging of the collateral ligaments is better evaluated using magnetic resonance imaging; however, it should be remembered that magic angle artefact and poor positioning can create many false positives (Spriet and Zwingenberger, 2009).
Cartilage imaging
Naturally occurring cartilage damage within the distal interphalangeal joint cannot be reliably detected on magnetic resonance imaging, whereas sharp sizeable defects in the articular cartilage can often be evident on low-field magnetic resonance imaging (van Zadelhoff et al, 2020). If a defect is seen on magnetic resonance imaging, it is likely to be real and indeed more extensive than depicted by imaging. It has been shown that the cartilage of the distal phalanx thins on low-field magnetic resonance imaging when a weight-bearing horse was compared to non-weight-bearing post mortem conditions, suggesting in vivo cartilage damage may be more difficult to identify (Evrard et al, 2019).
T2 mapping is a special magnetic resonance imaging sequence used in high-field magnets in humans to evaluate early cartilage proteoglycan loss in osteoarthritis. Preliminary proof of principle work using T2 mapping of the equine distal interphalangeal joint (with cartilage that is normally 2–3 mm thick) showed a good correlation between T2 maps and histology in cadaver limbs (Baker et al, 2023). Standing computed tomography can evaluate osteophyte presence and joint space narrowing (Figure 8), but further work is needed to determine the clinical relevance of these findings. Pauwels et al (2021) included a computed tomography arthrogram of the distal interphalangeal joint (Figure 9), and considered approximately 50% of the findings associated with this joint to be clinically significant. Imaging the cartilage of the metacarpophalangeal joint is much more challenging, as the cartilage is often <1 mm thick and this region is more susceptible to motion artefacts in the standing sedated patient.

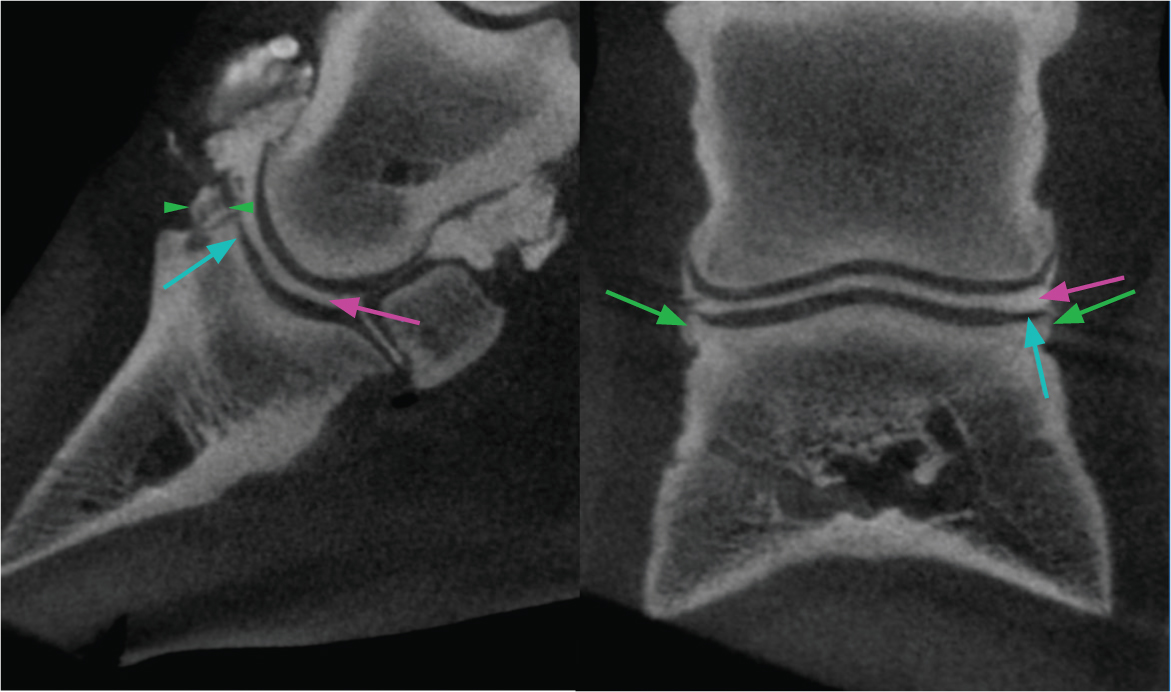
Conclusions
There is a growing body of evidence-based medicine that can guide clinicians and help selection of advanced imaging modality. Generally, computed tomography provides more information for the evaluation of cortical bone, and magnetic resonance imaging provides more information for the evaluation of soft tissues and disease activity. It should be remembered that the best image quality may not always be necessary to make an accurate clinical diagnosis, plan treatment and monitor rehabilitation. Furthermore, imaging findings must always be correlated to the clinical picture when determining the clinical significance of findings. Further studies comparing the sensitivity and specificity of the different available computed tomography and magnetic resonance imaging systems, ideally to the sensitivity and specificity of digital radiography, are needed. Such studies would allow the influences of updated motion correction software and the reduced slice thickness used currently in day-to-day practice to be assessed, and keep veterinarians informed on which systems are performing best for all the different conditions diagnosed within the equine distal limb. It is also important to determine which cases warrant the use multi-modal three-dimensional advanced imaging (both computed tomography and magnetic resonance imaging together), such that all cases are not ‘over-imaged’.
KEY POINTS
- In general, magnetic resonance imaging performs better for the diagnosis of tendon and ligament injury and the evaluation of bone oedema.
- Computed tomography performs better than low-field magnetic resonance imaging for the evaluation of cortical bone and the hoof wall because of the limited magnetic resonance imaging signal produced by these structures.
- Naturally occurring cartilage damage is often missed on low-field magnetic resonance imaging, but sharp defects in the cartilage can be visualised.
- Magnetic resonance imaging is capable of detecting bone oedema that may reflect ‘active’ changes, whereas dual-energy computed tomography that may be capable of detecting bone oedema has not yet been validated in the equine distal limb.
- Computed tomography imaging of the distal limb (foot–fetlock) can be performed in a short space of time (2–5 minutes), whereas low-field magnetic resonance imaging of the same region would take around 3 hours.