Horses are used in a variety of disciplines – including dressage, show jumping, eventing and racing – and the equine athlete is predisposed to the development of musculoskeletal injuries. Injury can be career limiting and present as a significant equine welfare concern. Poor performance can be the result of pain and the onset of lameness; however, riders and trainers may be unwilling to recognise behavioural changes as a manifestation of impaired welfare.
Equine osteoarthritis is a major cause of lameness, with over 60% of horses reported to develop osteoarthritis within their life-time (McIlwraith et al, 2012). However, its prevalence is thought to be much higher, with 80–90% of horses older than 15 years developing this chronic condition (Schlueter and Orth, 2004). It was first reported in 1938 and received clinical interest in 1966 at the American Association of Equine Practitioners. In 1975, it was established that cartilaginous lesions should act as objective criteria for the diagnosis of osteoarthritis (McIlwraith et al, 2012).
Clinical signs and symptoms of equine osteoarthritis
Today, it is understood that osteoarthritis is a disease of the entire joint, including cartilage, subchondral bone, ligaments, synovial membrane and periarticular tissues. It is recognised as incorporating multiple disorders characterised by a common end stage: the progressive deterioration of articular cartilage accompanied by changes in bone and soft tissue. As a disease of multifactorial origin, underlying factors include traumatic injury and idiopathic causes such as ageing. Osteoarthritis pathophysiology results in synovitis, cartilage degradation, osteophyte formation, subchondral bone sclerosis, fibrosis and reduced elastoviscosity of synovial fluid found within the joint capsule, as shown in Figure 1 (Clarke et al, 2021). Clinical diagnosis is founded upon radiographic and physical examination as well as reported history (Castanheira et al, 2021).
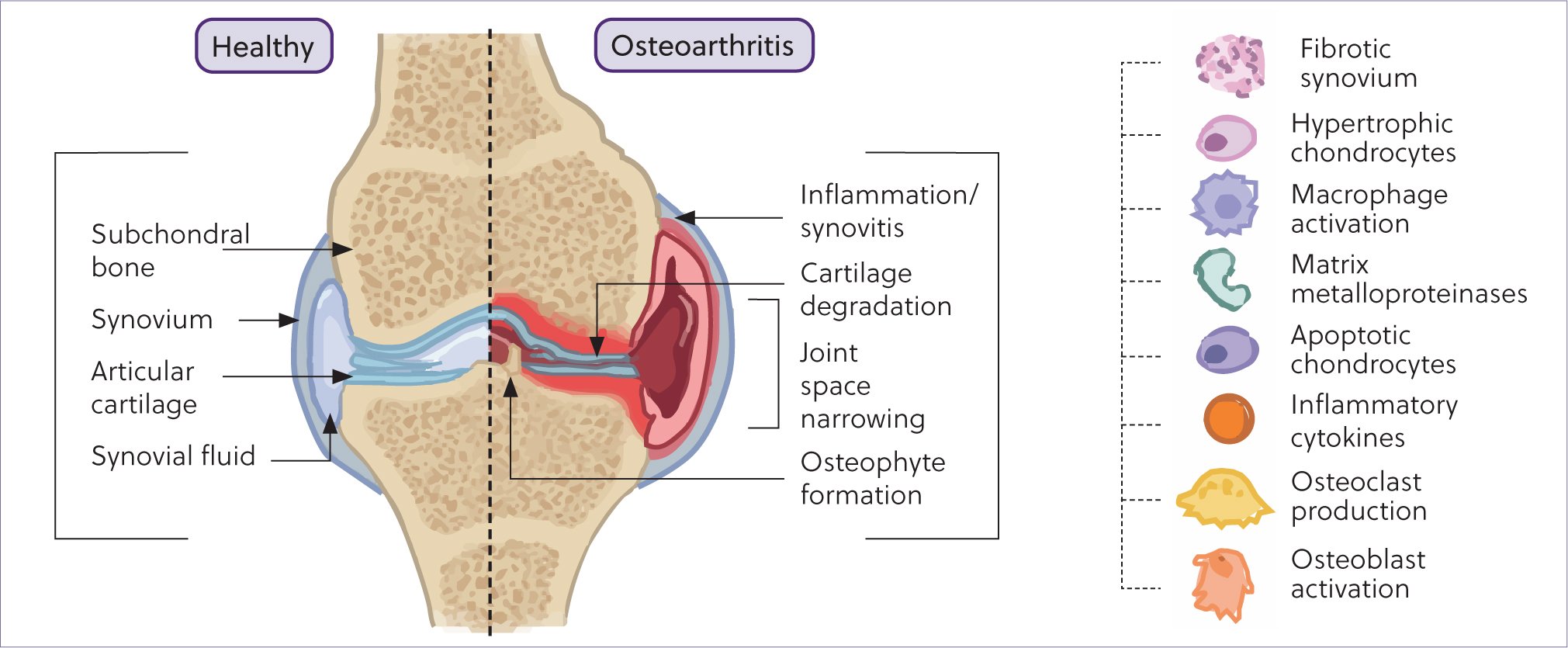
Osteoarthritis is regarded as a chronic degenerative disease taking many years to develop. Development can often be the result of trauma or injury as well as idiopathic causes such as ageing (Castanheira et al, 2021). In the horse, the risk of traumainduced osteoarthritis is often increased because of the athletic discipline the horse undertakes, with orthopaedic problems being the primary cause of loss of use and death in athletic horses. This results in more than 70% of days lost to training in both show jumpers and racehorses (Ribitsch et al, 2021). Factors that may predispose a horse to osteoarthritis include age, mechanical injury, genetics, gender, metabolic dysfunction and obesity (Ribitsch et al, 2021).
Molecular hallmarks of equine osteoarthritis
Osteoarthritis is characterised by a range of molecular changes within the joint resulting in the cascade of cartilage destruction and impairment of the microenvironment. These include a reduction of glycosaminoglycans and increased production of mediators of homeostatic processes within the joint, such as matrix metalloproteinases, aggrecanases, prostaglandins, free radicals and cytokines including interleukin-1β and tumour necrosis factor-α (McIlwraith et al, 2012). It has been previously demonstrated that tumour necrosis factor-α can be found in greater abundance in the synovium, synovial fluid and cartilage of equine osteoarthritic carpi, and interleukin-1β is expressed in significantly greater amounts in osteoarthritic cartilage (Kamm et al, 2010). Thus, interleukin-1β has been referred to as the master cytokine in human osteoarthritis and this finding is conserved across species (Rivers-Auty et al, 2018; Deng et al, 2021; Watkins et al, 2021). In addition, the increase of chemokines promotes inflammation, resulting in the recruitment of inflammatory cells to bone, connective tissues and cartilage.
Extracellular vesicles
A brief history of extracellular vesicles
Extracellular vesicles are nanoparticles secreted by most mammalian cells. They are enveloped in a phospholipid bilayer membrane and are unable to replicate. They were first identified as biological entities with enzymatic and functional potential in the 1980s and 1990s. Rowbotham (1980) and Matsuyama et al (1986) are examples of some of the earliest manuscripts referring to extracellular vesicles. Some of the earliest experiments involving extracellular vesicles were undertaken by Chargraff and West in the 1940s while studying blood coagulation (Chargraff and West, 1946; Couch et al, 2021). Chargaff and West described coagulant lipoproteins with a high particle weight that were separated from platelets by differential centrifugation – unknown to them, this was the first documentation of extracellular vesicles. Peter Wolf identified ‘material in minute particulate form, sedimentable by high-speed centrifugation and originating from platelets, but distinguishable from intact platelet’ 17 years later, and referred to as it ‘platelet dust’ (Wolf, 1967; Couch et al, 2021).
Extracellular vesicle characterisation
As per the Minimal Information for Studies of Extracellular Vesicles 2018 guidelines (Théry et al, 2018), isolated extracellular vesicles must be characterised in order to demonstrate a sample comprises of extracellular vesicles, because of their size. It is a requirement under these guidelines that extracellular vesicles are characterised using global quantification methods, such as protein content, particle number, lipid content, or transmembrane or cytosolic proteins. In addition, it is suggested that an assessment of contaminants should be conducted. Images of extracellular vesicles are recommended to be taken using electron microscopy, scanning probe microscopy or super resolution fluorescence microscopy. Non-image-based analysis of large numbers of extracellular vesicles should also be used, for example nanoparticle tracking analysis and flow cytometry (Théry et al, 2018). Transmission electron microscopy is a popular form of extracellular vesicle characterisation, and has a resolution of a nanometre. It has the capacity to distinguish extracllular vesicles from similar sized particles. However, there is a need to improve reproducibility and comparability of this method and eliminate bias in operator image selection (Rikkert et al, 2019). Nanoparticle tracking analysis uses light scattering and the Brownian motion of particles in order to provide rapid sizing and enumeration of vesicles. In addition, the temperature and viscosity of the sample is considered and applied to the Stokes–Einstein equation.
Extracellular vesicle biology
These nanoparticles are involved in intercellular communication, by transporting biological cargo between cells (Cocucci et al, 2015; Konoshenko et al, 2018; Battistelli and Falcieri, 2020). This has been defined by the International Society of Extracellular Vesicles within their minimal information for studies involving extracellular vesicles, first in 2014 and updated in 2018 (Théry et al, 2018). Extracellular vesicles can be categorised by size, density and biochemical composition. Broadly, extracellular vesicles are subdivided into three groups, as shown in Figure 2.
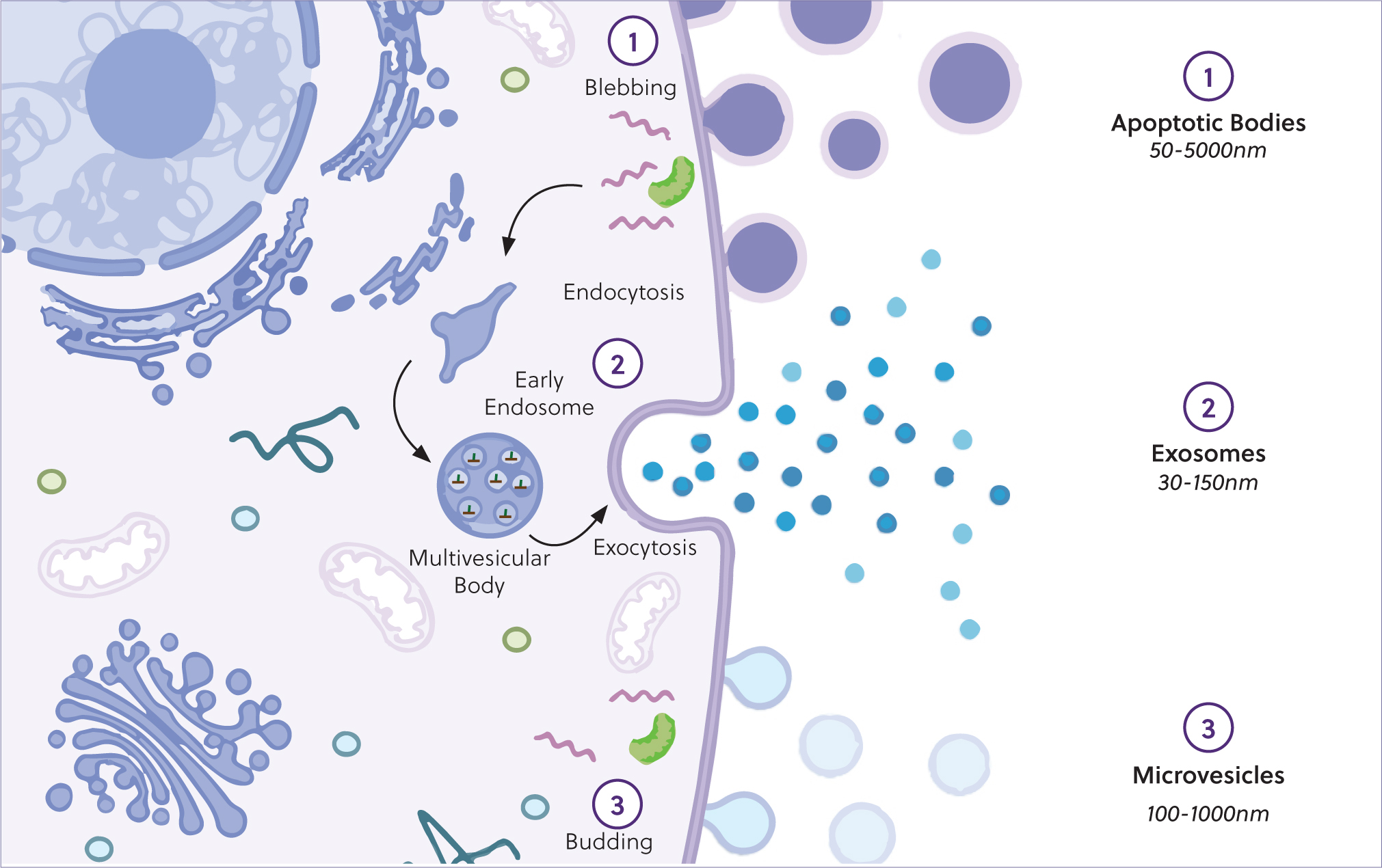
Exosomes are the smallest subtype of extracellular vesicles, with a diameter of 40–120 nm. They are formed as intraluminal vesicles during the assembly of multivesicular bodies. These vesicles are present in most biological fluids and can serve as a source for potential biomarker discovery. The protein and lipid composition of exosomes reflect cellular origin, with the most common proteins being annexins, tetraspanins (CD63, CD81, CD82 and CD9) and heat-shock proteins (HSP60, HSP70 and HSP90) (Battistelli and Falcieri, 2020; Mathieu et al, 2021). Extracellular vesicle cargo is dependent on the cellular source and extracellular vesicle subtype. Relevant studies probing the cargo of extracellular vesicles are discussed in the following sections with respect to disease pathology, and the mechanisms of biological therapeutics, as well as engineered cargo delivery systems.
Microvesicles are the second largest form of vesicles and are formed from the direct budding of the cell membrane, with a diameter of 100–500 nm in size. These also carry proteins, nucleic acids and bioactive lipids of the cell of origin, and often express surface markers reflective of the cellular origin. Both these subtypes of extracellular vesicles induce phenotypical and functional changes, relevant in several physio-pathological conditions (Battistelli and Falcieri, 2020).
Last, cells undergoing apoptosis secrete apoptotic bodies, that have a diameter of 500 nm–2 µm. With respect to biomarker discovery, disease pathogenesis and mediation of biological therapies, exosomes and microvesicles are the most relevant.
Extracellular vesicles in equine osteoarthritis
Extracellular vesicles have been implicated in the pathogenesis of osteoarthritis and hypothesised to contain biomarkers that may serve as targets for diagnostic tests. All joint tissues and associated structures, including the synovium (synoviocytes), cartilage (chondrocytes), subchondral bone (osteoblasts, osteocytes and osteoclasts) and tendon (tenocytes) secrete extracellular vesicles (Ni et al, 2020). As such, it has been suggested that extracellular vesicles regulate joint homeostasis and regeneration in articular tissues (Boere et al, 2018; Herrmann et al, 2021; Mustonen and Nieminen, 2021). In osteoarthritis, it has been postulated and subsequently demonstrated that extracellular vesicles can propagate disease through the joint by transporting and enhancing the production of inflammatory mediators and cartilage-degrading proteinases (Mustonen and Nieminen, 2021).
In vitro studies
In vitro studies have been conducted across a range of species, including humans, when exploring the role of extracellular vesicles in osteoarthritis, forming the basis of understanding of extra-cellular vesicle biology in osteoarthritis. Liu et al (2020) explored the role of chondrocyte-derived extracellular vesicles in vitro, and found that extracellular vesicles from human chondrocytes stimulated by interleukin-1β inhibited chondrogenesis. Ni et al (2020) explored the effect of stimulated chondrocytes in humans, and identified that extracellular vesicles from osteoarthritic chondrocytes increased interleukin-1β production in macrophages, resulting in decreased autophagy and the production of mitochondrial reactive oxygen species (Ni et al, 2019). It is important to note that if extracellular vesicles and their subsequent cargo are to be used as biomarkers of disease, they must be useful in identifying early osteoarthritis in order to have a prognostic value. Therefore, further studies are required using early osteoarthritis phenotypes.
In vivo studies
A number of in vivo studies have been performed exploring the role of extracellular vesicles in the pathogenesis of equine osteoarthritis throughout the articular joint. These include the use of large animal experimental models or clinical cohorts. A study by Mustonen et al (2021) used synovial fluid collected from the metacarpophalangeal joints following euthanasia, and identified that the number of extracellular vesicles containing hyaluronic acid decreased with osteoarthritis and may serve as a biomarker for joint disease. However, further investigation is required to determine their role in disease pathogenesis. In addition, Mustonen et al (2023) quantified unique fatty acid signatures in synovial fluid-derived extracellular vesicles. These fatty acid signatures included palmitic acid, stearic acid and behenic acid, and are known to play a role in the inflammatory processes and cartilage degradation in osteoarthritis (Mustonen et al, 2023). Further to this, Anderson et al (2022) probed plasma and synovial fluid-derived extracellular vesicles from horses enrolled in a carpal osteochondral fragment model, a known temporal experimental model of osteoarthritis. Following transcriptomic analysis of extracellular vesicles derived from biofluids taken longitudinally over a 70-day period of disease development, there were temporal expression changes of seven small non-coding RNAs called microRNAs observed from both plasma and synovial fluid. These were:
- eca-miR-451
- eca-miR-25
- eca-miR-215
- eca-miR-92a
- eca-miR-let-7c
- eca-miR-486-5p
- eca-miR-23a.
In addition, four small nucleolar RNAs (snoRNAs) – U3, snord15, snord46 and snord58 – were also differentially expressed. Using computational methods to determine the role of the micro-RNAs in osteoarthritis functional enrichment analysis identified that they were involved in inhibition of the cell cycle, cell proliferation and DNA damage (Anderson et al, 2022). Last, Clarke et al (2022a) conducted a study exploring the metabolomic composition of plasma-derived extracellular vesicles from a clinical cohort of thoroughbred racehorses. Using infrared spectroscopy, plasma-derived extracellular vesicles from horses diagnosed with osteoar-thritis showed a significantly increased signal compared to healthy animals with respect to protein, lipids and nucleic acids (Clarke et al, 2022a). Increased signal in this context is synonymous with an increased amount of said molecule with a given experimental group, and if significant following statistical analysis this can be attributed as a unique characteristic of this group.
Further studies have probed their cargo and capacity to serve as biomarkers: for example fatty acid signature have been identified in extracellular vesicles from bronchoalveolar lavage fluid as an indicator of equine asthma severity (Höglund et al, 2023). Further to this, studies have evaluated the role of plasma extracellular vesicles diagnostically. de Oliveira et al (2021) explored miRNA content and expression variation longitudinally in equines in order to explore the effect of endurance racing. Thus, they could potentially be used as a markers of health status. Another study identified cell-specific plasma microvesicles that varied with season and disease predisposition in healthy and previously laminitic ponies, which has significant implications for equine health and disease management (Finding et al, 2018) and highlights the capacity of plasma-derived extracellular vesicles to be used diagnostically in equine medicine.
Extracellular vesicles in the treatment of equine osteoarthritis
Targeted drug delivery
During recent years, extracellular vesicles have become an attractive and viable drug delivery system for a range of diseases (Escudé Martinez de Castilla et al, 2021). Extracellular vesicles have the capacity to selectively and specifically transfer their biological contents between donor and recipient cells (Liang et al, 2021), with the ability to have their cargo biologically engineered and enriched to improve the efficacy of a given treatment. As such, a number of studies have been performed across a range of species and diseases, including cancers and cardiovascular disease to name just a few (Hafiane and Daskalopoulou, 2018; Liu et al, 2019).
In osteoarthritis, it has been demonstrated that intra-articular administration of chondrocyte-derived extracellular vesicles can restore mitochondrial function (Liang et al, 2021). Moreover, it has been shown that the target delivery of genetically engineered exosomes enriched in microRNA-140 in a rat model of osteoarthritis resulted in improved delivery of miR-140 to deep cartilage regions, inhibiting cartilage-degrading proteases, and alleviated osteoarthritis progression in a rat model, following chondrocyte affinity peptide fusion to the surface of enriched extracellular vesicles (Liang et al, 2020). In addition, it has been demonstrated that extra-cellular vesicles derived from miR-140-5p-overexpressing synovial mesenchymal stem cells have the capacity to prevent an osteoar-thritis phenotype by enhancing the proliferation and migration of articular chondrocytes, without inhibiting extracellular matrix secretion, both in vitro and in vivo in a rat model of osteoarthritis (Tao et al, 2017). Hence, although in its infancy, research is moving towards exploring the potential of extracellular vesicles as drug delivery systems in the context of osteoarthritis in order to provide a targeted form of treatment that can be translated across a range of species including equines.
Mesenchymal stromal cell therapy
Mesenchymal stromal cells have been a prolific source of potential for regenerative therapies and are used routinely in the treatment of orthopaedic pathologies in veterinary practice, including equine osteoarthritis. However, their use harbours inherent risks, namely (Hotham and Henson, 2020):
- Uncontrollable proliferation
- Migration
- Immune response initiation
- Invasive surgery
- Cellular expansion time
- Delivery of cells in a suitable suspension for injection.
In addition, the discourse surrounding the mechanistic action of mesenchymal stromal cells has altered, in favour of a secretome and extracellular vesicle-mediated effect (D'Arrigo et al, 2019). Mesenchymal stromal cell-derived extracellular vesicles have become a popular area of investigation in the realm of cell-free biological therapeutics, as they offer a safer alternative to cell-based therapeutics, while alleviating the effect of factors such as cell ageing, disadvantageous immune response and inflammation. As extracellular vesicles have the capacity to carry bioengineered biological cargo, this offers greater control over delivered bioactive materials in order to maximise therapeutic potential (Boulestreau et al, 2020; Racchetti and Meldolesi, 2021). Mesenchymal stromal cell-derived extracellular vesicles have been shown to modulate immune reactivity, promote angiogenesis and accelerate cell proliferation and migration through cell signalling pathways. Specifically in osteoarthritis, they have been shown to promote cartilage repair, inhibit synovitis and mediate subchondral bone remodelling (Ni et al, 2020). Thus, they encompass many of the same properties as the ‘parent’ mesenchymal stromal cell (Zhu et al, 2020). However, they are more stable, adaptable and have a high cellular affinity. Furthermore, their regenerative capacity has been observed in a number of systems including the skin, heart, kidney, central and peripheral nerves, as well as musculoskeletal tissues such as cartilage, tendon, ligament and bone (Zhu et al, 2020).
Specifically in the context of equine osteoarthritis, several in vitro and in vivo studies have been performed to explore the mechanisms of mesenchymal stromal cell-derived extracellular vesicle-based therapeutics. Hotham et al (2021) stimulated autologous equine chondrocytes with tumour necrosis factor-α and interleukin-1β in order to produce an inflammatory osteoarthritis phenotype. Stimulated cells were then treated with bone marrow-derived mesenchymal stromal cell-derived extracellular vesicles. These extracellular vesicles were incorporated into cells and had an anti-inflammatory effect (Hotham et al, 2021). A similar study was performed by Arévalo-Turrubiarte et al (2022), validating these previous findings, while also identifying a significant decrease in metalloproteinase-13 expression in stimulated equine chondrocytes. In vivo studies have also been performed using an equine in vivo model of synovitis, induced using 0.25 ng of lipopolysac-charide into the radiocarpal joint. This study compared the use of interferon gamma and tumour necrosis factor-α-stimulated, allogenic equine bone marrow-derived mesenchymal stromal cell treatment to mesenchymal stromal call secretome treatment containing extracellular vesicles. It found that the mesenchymal stromal cell secretome significantly reduced joint circumference and increased the concentration of glycosaminoglycans. In addition, no significant difference was observed between mesenchymal stromal cell secretome treatment and mesenchymal stromal cell treatment (Kearney et al, 2022), indicating that mesenchymal stromal cell secretome could be a viable off-the-shelf alternative to mesenchymal stromal cell treatment of orthopaedic diseases such as osteoarthritis.
Studies are already underway to determine the properties of secretome based products and the scaling up of therapeutic product development. For example, an intra-articular injectable pharmaceutical powder has been formulated, derived from the equine mesenchyman stromal cell secretome, and the technological process has been validated in an authorised facility for veterinary clinical-use medicinal production by Mocchi et al (2020).
Future perspectives
It has become apparent that extracellular vesicles have the capacity to transport biologically relevant cargo and can facilitate the progression of an osteoarthritis phenotype within the articular joint. Extracellular vesicles from both plasma and synovial fluid have the capacity to serve as relevant sources for diagnostically applicable biomarkers in the future. These biomarkers could also serve as potential targets for the development of novel therapeutics. However, necessary considerations must be made in order for extracellular vesicles to translate effectively to clinical practice, such as the sensitivity of equipment being used to analyse extracellular vesicles and the efficacy of extracellular vesicle isolation methods (Clarke et al, 2022a). Current studies exhibit a diverse range of characterisation and isolation procedures which will result in extracellular vesicle heterogeneity (Brien and Hollinshead, 2023). Therefore, more stringent standardisation is crucial.
In addition, as extracellular vesicle-based therapeutics are explored further in the context of joint disease, research is required to identify the most optimal cellular sources (Clarke et al, 2022b), extracellular vesicle biodistribution and therapeutic delivery methods (Wiklander et al, 2015; Brien and Hollinshead, 2023), to facilitate extracellular vesicle-mediated restoration of joint homeostasis (Boere et al, 2018). This could, in the future, offer an effective ‘off the shelf’ cell-free therapeutic for joint disease, although the research required into scale up and manufacturing of extracellular vesicles from culture-expanded mesenchymal stromal cells should not be underestimated.
KEY POINTS
- Equine osteoarthritis is a significant equine welfare concern, and currently there are no diagnostic biomarkers to identify early osteoarthritis, nor any curative treatments.
- Extracellular vesicles are nanoparticles secreted by most mammalian cells and are an emerging source of biomarkers in disease.
- Extracellular vesicles have the capacity to serve as a cell-free therapeutic when derived from specific sources, such as mesenchymal stromal cells.
- Extracellular vesicles are still an emerging field of research and work is required to make them clinically translatable. Research is currently being conducted in order to tackle these issues.